Practical Medicinal Chemistry with Macrocycles
Design, Synthesis, and Case Studies
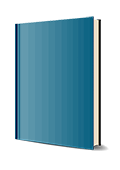
1. Auflage September 2017
624 Seiten, Hardcover
Wiley & Sons Ltd
Kurzbeschreibung
Including case studies of macrocyclic marketed drugs and macrocycles in drug development, this book helps medicinal chemists deal with the synthetic and conceptual challenges of macrocycles in drug discovery efforts. With chapters contributed from leading international figures involved in macrocyclic drug discovery efforts, the book gives readers the required background, expertise and case studies to build a program in macrocycle drug discovery - including design criteria, typical profile of current macrocycles, applications, and limitations.
Including case studies of macrocyclic marketed drugs and macrocycles in drug development, this book helps medicinal chemists deal with the synthetic and conceptual challenges of macrocycles in drug discovery efforts.
* Provides needed background to build a program in macrocycle drug discovery -design criteria, macrocycle profiles, applications, and limitations
* Features chapters contributed from leading international figures involved in macrocyclic drug discovery efforts
* Covers design criteria, typical profile of current macrocycles, applications, and limitations
Introduction
About the contributors
Part I Challenges Specific to Macrocycles
1. Contemporary Macrocyclization Technologies
Serge Zaretsky and Andrei K. Yudin
1.1. Introduction
1.2. Challenges inherent to the synthesis of macrocycles
1.3. Challenges in macrocycle characterization
1.4. Macrocyclization methods
1.5. Cyclization on the solid phase
1.6. Summary
1.7. References
2. A Practical Guide to Structural Aspects of Macrocycles (NMR, X-Ray and Modelling)
David J. Craik, Quentin Kaas and Conan K. Wang
Abstract
2.1. Background
2.1.1. Classes of macrocycles covered
2.1.2. Applications of macromolecules in drug design and agriculture and the role of structural information in these applications
2.1.3. Experimental techniques (NMR and X-ray)
2.1.4. Modelling studies
2.2. Experimental studies of macrocycles
2.2.1. NMR experiments and parameters that yield structural information
2.2.2. Protocols for 3D structural determination using NMR
2.2.3. Dynamic aspects of structures (NMR relaxation)
2.2.4. X-ray studies of macrocycles
2.2.5. Macrocycle-receptor interactions (both NMR and X-ray)
2.3. Molecular modelling of macrocyclic peptides
2.3.1. Methods and challenges in modelling cyclic peptides
2.3.1.1. Quantum mechanics
2.3.1.2. Molecular mechanics
2.3.2. Conformation, dynamics and electrostatics of cyclic peptides
2.3.2.1. NMR spectroscopy combined with MD simulations
2.3.2.2. Studying large conformational ensembles and folding
2.3.2.3. Electrostatic characteristics of cyclic peptides
2.3.3. Modelling the activity of cyclic peptides
2.3.3.1. Cyclic peptide interactions with molecular targets
2.3.3.2. Cyclic peptide nanotubes
2.3.3.3. Membrane permeation and diffusion
2.3.4. Engineering cyclic peptides as grafting scaffolds
2.4. Summary
2.5. Acknowledgments
2.6. List of abbreviations
2.7. References
3. Designing Orally Bioavailable Peptide and Peptoid Macrocycles
David Price, Alan M. Mathiowetz and Spiros Liras
3.1. Introduction
3.2. Improving peptide plasma half-life
3.3. Absorption, bioavailability and methods for predicting absorption
3.3.1. In vitro assays
3.3.2. Paracellular absorption
3.3.3. Tight junction modifiers to improve paracellular absorption
3.3.4. Transcellular absorption of macrocycles
3.3.4.1. Cyclisation
3.3.4.2. N-methylation
3.3.4.3. Cyclosporine A
3.3.4.4. Conformational interconversion and H-bond networks
3.3.4.5. Shielding
3.3.4.6. Additional strategies for managing H-bond networks
3.4. In silico modeling
3.5. Future directions
3.6. References
Part II Classes of Macrocycles and their Potential for Drug Discovery
4. Natural and Nature-Inspired Macrocycles - A Chemoinformatic Overview and Relevant Examples
Ludger A. Wessjohann, Richard Bartelt, Ricardo A. W. Neves Filho,
Wolfgang Brandt
4.1. Introduction to natural macrocycles as drugs and drug leads
4.2. Biosynthetic pathways, natural role and biotechnological access
4.3. QSAR and chemoinformatic analyses of common features
4.4. Case studies: selected natural macrocycles of special relevance in medicinal chemistry
4.5. References
5. Bioactive and Membrane-Permeable Cyclic Peptide Natural Products
Andrew T. Bockus and R. Scott Lokey
5.1. Introduction
5.2. Structural motifs and permeability of cyclic peptide natural products
5.3. Conformations of passively permeable bioactive cyclic peptide natural products
5.3.1. Flexible scaffolds
5.3.2. Structural analogs
5.3.3. Lipophilic (AlogP > 3) peptides and reported bioactivities
5.4. Recently discovered bioactive cyclic peptide natural products
5.4.1. Mid-Sized Macrocycles
5.4.1.1. Cytotoxics
5.4.1.2. Antibacterials
5.4.1.3. Antivirals
5.4.1.4. Antiparasitics
5.4.1.5. Antifungals
5.4.1.6. Protease Inhibitors
5.4.1.7. Other Bioactivities
5.4.2. Large/Complex Peptides
5.4.2.1. Cystine knots
5.4.2.2. Lantibiotics
5.5. Conclusions
5.6. References
6. Chemical Approaches to Macrocycle Libraries
Ziqing Qian, Patrick G. Douherty, Dehua Pei
6.1. Introduction
6.2. Challenges Associated with Macrocyclic OBOC Libraries
6.3. Deconvolution of Macrocyclic Libraries
6.4. Peptide-Encoded Macrocyclic Libraries
6.5. DNA-Encoded Macrocyclic Libraries
6.6. Parallel Synthesis of Macrocyclic Libraries
6.7. Diversity-Oriented Synthesis
6.8. Perspective
6.9. Conclusion
6.10. References
7. Biological and Hybrid Biological/Chemical Strategies in Diversity Generation of Peptidic Macrocycles
Francesca Vitali & Rudi Fasan
7.1. Introduction
7.2. Cyclic peptide libraries on phage particles
7.2.1. Disulfide-bridged cyclic peptide libraries
7.2.2. From phage display to peptide macrocycle design
7.2.3. Bicyclic peptide libraries on phage
7.3. Macrocyclic peptide libraries via in vitro translation
7.3.1. In vitro cyclic peptide libraries via chemical crosslinking
7.3.2. In vitro macrocyclic peptide libraries via the FIT and RaPID system
7.4. Emerging strategies for the combinatorial synthesis of hybrid macrocycles in vitro and in cells.
7.4.1. Macrocyclic Organo-Peptide Hybrids (MOrPHs).
7.4.2. Synthesis of macrocyclic peptides in living cells.
7.5. Comparative analysis of technologies
7.6. Conclusion
7.7. References
8. Macrocycles for Protein-Protein Interactions
Eilidh Leitch & Ali Tavassoli
8.1. Introduction
8.2. Library Approaches to Macrocyclic PPI Inhibitors
8.2.1. SICLOPPS
8.2.2. FIT and RaPID
8.3. Structural Mimicry
8.3.1. ß-strands
8.3.2. alpha-helices
8.4. Multi-cycles for PPIs
8.5. The Future for Targeting PPIs with Macrocycles
8.6. List of Abbreviations
8.7. References
Part III The Synthetic Toolbox for Macrocycles
9. Synthetic Strategies for Macrocyclic Peptides
Éric Biron, Simon Vézina-Dawood, François Bédard
9.1. Introduction: peptide macrocyclization
9.1.1. Cyclic peptide topologies
9.1.2. Solution phase vs solid-supported macrocyclizations
9.2. One size does not fit all: factors to consider during synthesis design
9.2.1. Ring size
9.2.2. Incorporation of turn-inducing elements
9.2.3. C-terminus epimerization
9.2.4. Choosing the right macrocyclization site
9.3. Peptide macrocyclization in solution
9.3.1. Ring contraction strategies
9.3.2. Sulfur-mediated macrocyclizations
9.3.3. Cyclic depsipeptides and peptoids
9.4. Peptide Macrocyclization on solid support
9.4.1. Side chain anchoring
9.4.2. Backbone amide anchoring
9.4.3. Safety-catch anchoring and cyclative cleavage
9.5. Peptide Macrocyclization by disulfide bond formation
9.5.1. Disulfide bond formation in solution
9.5.2. Disulfide bond formation on solid support
9.6. Conclusion
10. Ring Closing Metathesis-Based Methods in Chemical Biology: Building a Natural Product-Inspired Macrocyclic Toolbox to Tackle Protein-Protein Interactions
Jagan Gaddam, Naveen Kumar Mallurwar, Saidulu Konda, Mahender Khatravath, Madhu
Aeluri, Prasenjit Mitra and Prabhat Arya
10.1. Introduction
10.2. Protein-Protein Interactions - Challenges and Opportunities
10.3. Natural Products as Modulators of Protein-Protein Interactions
10.4. Introduction to Ring Closing Metathesis (RCM)
10.4.1. Ring Closing Olefin Metathesis
10.4.2. Z-Selective Ring Closing Metathesis
10.5. Selected Examples of Synthetic Macrocyclic Probes using RCM Based Approaches
10.5.1. Identification of Sonic Hedgehog Inhibitor from the RCM Library
10.5.2. Identification of Anti-Malarial Compounds from the RCM Library
10.5.3. Synthesis of Natural Product-Like Molecules Using RCM as the Key Strate
10.5.4. Alkaloid Natural Product-Inspired Macrocyclic Chemical Probes
10.5.5. Indoline Alkaloid-Inspired Macrocyclic Chemical Probes
10.5.6. Tetrahydroquinoline Alkaloid-like Macrocyclic Chemical Probes
10.5.7. Enantio-enriched, Benzofuran-based, Macrocyclic Toolbox
10.5.8. Building a Diverse 14-Membered Ring-based Chemical Toolbox
10.5.9. Building a Diverse C-Linked Glyco-based Macrocyclic Toolbox
10.5.10. Evaluation of the chemical toolbox to search for anti-angiogenesis agents
10.6. Summary
10.7. References
11. The Synthesis of Macrocycles by Huisgen Cycloaddition
Ashok D. Pehere and Andrew D. Abell
11.1. Introduction
11.2. Dipolar cycloaddition reactions
11.3. Macrocyclic peptidomimetics
11.3.1. Macrocyclic antagonists for the treatment of cancer
11.3.2. Dimeric macrocyclic antagonists of apoptosis proteins
11.3.3. Macrocyclic Grb2 SH2 domain inhibitors
11.3.4. STAT3 inhibitors
11.3.5. Histone deacetylase inhibitors
11.3.6. Somatostatin modulators
11.4. Macrocyclic?n?Ò-strand mimetics as protease inhibitors
11.5. Conclusion
11.6. References
12. Palladium-catalysed synthesis of macrocycles
Thomas O. Ronson, William P. Unsworth and Ian J. S. Fairlamb
12.1. Introduction
12.2. Stille Reaction
12.3. Suzuki-Miyaura reaction
12.4. Heck reaction
12.5. Sonogashira reaction
12.6. Tsuji-Trost reaction
12.7. Other reactions
12.8. Conclusion
12.9. References
13. Alternative Strategies for the Construction of Macrocycles
Jeffrey Santandrea, Anne-Catherine Bédard, Mylène de Léséleuc, Michael Raymond and Shawn K. Collins
13.1. Introduction
13.2. Alternative methods for macrocyclization involving Carbon-Carbon bond formation
13.2.1. Alkylation
13.2.2. Glaser-Hay coupling
13.2.3. Nickel /Ruthenium/Copper-catalyzed couplings
13.2.4. Wittig and other olefinations
13.2.5. Cyclopropanation
13.2.6. Oxidative coupling of arenes
13.2.7. Gold catalysis
13.3. Alternative methods for macrocyclization involving carbon-carbon bond formation: ring expansion and photochemical methods
13.3.1. Ring expansion
13.3.2. Photochemical methods
13.4. Alternative methods for macrocyclization involving carbon-oxygen bond formation.
13.4.1. Cham-Lam-Evans coupling
13.4.2. Alkylation
13.4.3. Nucleophilic aromatic substitution
13.4.4. Ullmann coupling
13.4.5. C-H activation
13.5. Alternative methods for macrocyclization involving carbon-nitrogen bond formation.
13.5.1. Alkylation
13.5.2. Nucleophilic aromatic substitution
13.5.3. Ullmann coupling
13.6. Alternative methods for macrocyclization involving carbon-sulfur bond formation.
13.6.1. Ramberg-Bäcklund chemistry
13.6.2. Thiol-ene reaction
13.7. Conclusion and summary
13.8. References
14. Macrocycles from Multicomponent Reactions
Ludger A. Wessjohann, Ricardo A. W. Neves Filho, Alfredo R. Puentes, Micjel C. Morejon
14.1. Introduction
14.2. General Aspects of Multicomponent Reactions (MCRs) in Macrocycle Syntheses
14.2.1. The MiB Concept
14.2.2. Unidirectional Multicomponent Macrocyclizations / Cyclooligomerizations
14.2.3. Bidirectional Multicomponent Macrocyclizations
14.2.4. Iterative IMCR-based macrocyclizations with multiple bifunctional building blocks
14.3. Concluding remarks and future perspectives
14.4. References
15. Synthetic Approaches Used in the Scale-up of Macrocyclic Clinical Candidates
Jonrock Kong
15.1. Introduction
15.2. Background
15.3. Literature Examples
15.3.1. Macrolactonization
15.3.2. Macrolactamization
15.3.3. Ring-closing Metathesis (RCM)
15.3.4. Metal-Catalyzed Cross-Coupling
15.3.5. Oxidative Disulfide Formation
15.3.6. Other Approaches
15.4. Conclusions
15.5. References
Part IV Macrocycles in Drug Development - Case Studies
16. Overview of Macrocycles in Clinical Development and Clinically Used
Silvia Stotani and Fabrizio Giordanetto
16.1. Introduction
16.2. Datasets generation
16.3. Marketed macrocyclic drugs
16.3.1. General characteristics
16.3.2. Cyclic peptides
16.3.3. Macrolides and ansamycins
16.3.4. Bioavailability and doses of macrocyclic drugs
16.4. Macrocycles in clinical studies
16.4.1. General characteristics
16.4.2. Cyclic peptides
16.4.3. Macrolides and ansamycins
16.5. De novo designed macrocycles
16.5.1. Protease and polymerase inhibitors
16.5.2. Kinase inhibitors
16.6. Overview and conclusions
16.7. List of abbreviations
16.8. References
17. The Discovery of Macrocyclic IAP Inhibitors for the Treatment of Cancer
Nicholas K. Terrett
17.1. Introduction
17.2. DNA-programmed chemistry macrocycle libraries
17.2.1. Initial IAP screening macrocycle hits
17.2.2. A follow-up DPC macrocycle library
17.3. A new macrocycle ring structure
17.3.1. Functional caspase-3 rescue assay
17.4. Design and profiling of bivalent macrocycles
17.4.1. In vitro anti-proliferative activity
17.4.2. Pharmacokinetic profiling
17.4.3. In vivo efficacy in a xenograft model
17.5. Improving the profile of bivalent macrocycles
17.5.1. Replacing carboxylic acids
17.5.2. Replacing triazole linkers
17.6. Selection of the optimal bivalent macrocyclic IAP antagonist
17.6.1. Synthesis of the optimal bivalent macrocycle
17.6.2. In vitro profiling
17.6.3. Pharmacokinetic profiling
17.6.4. In vivo efficacy in a xenograft model
17.7. Summary
17.8. References
18. Discovery and Pharmacokinetic-Pharmacodynamic Evaluation of an Orally Available Novel Macrocyclic Inhibitor of Anaplastic Lymphoma Kinase and cRos Oncogene 1
Shinji Yamazaki, Justine L. Lam, Ted W. Johnson
18.1. Introduction
18.2. Discovery and Synthesis
18.2.1. Background - Macrocyclic kinase inhibitors
18.2.2. Crizotinib Discovery and SAR
18.2.3. Resistance Mechanisms to Crizotinib
18.2.4. Program Goals and Lab Objectives
18.2.5. Crizotinib and PF-06439015 - structural data, potency, ADME
18.2.6. Acyclic ALK inhibitors
18.2.7. Design from Acyclic Structural Data
18.2.8. Macrocyclic ALK inhibitors
18.2.9. Selectivity Strategy
18.2.10. Structural Analysis of PF 06463922
18.2.11. Overlapping potency and selectivity
18.2.12. Synthesis of PF 06463922
18.2.13. Summary of Discovery and Synthesis
18.3. Evaluation of Pharmacokinetic Properties including CNS Penetration
18.3.1. Background
18.3.2. Lab Objectives and In Vitro Screening for CNS Penetration
18.3.3. ADME Evaluation
18.3.4. In Vivo Assessment of Brain Penetration in Rats Measuring Brain Homogenate and CSF
18.3.5. In Vivo Assessment of Brain Penetration in Rats using Quantitative Autoradiography
18.3.6. In Vivo Assessment of Spatial Brain Distribution in Mice using Matrix-Assisted Laser Desorption Ionization-Mass Spectrometry Imaging
18.3.7. In Vivo Efficacy Assessment of Orthotopic Brain Tumor Model using Magnetic Resonance Imaging
18.3.8. PK and Brain Penetration Summary
18.4. Evaluation of Pharmacokinetic-Pharmacodynamic (PKPD) Profiles
18.4.1. Background
18.4.2. In Vivo Nonclinical Studies
18.4.3. PK Modelling
18.4.4. PKPD Modelling for Target Modulation
18.4.5. PKDZ Modelling for Antitumor Efficacy
18.4.6. Quantitative Comparison of Exposure-Response Relationships
18.4.7. PKPD Summary
18.5. Conclusion
18.6. List of Abbreviations
18.7. References
19. Optimization of a Macrocyclic Ghrelin Receptor Agonist (Part II): Development of TZP-102
Hamid R. Hoveyda, Graeme L. Fraser, Eric Marsault, René Gagnon and Mark L. Peterson
19.1. Introduction
19.2. Advanced AA3 and tether SAR
19.2.1. AA3 options for improved CYP3A4 profile
19.2.2. Additional Tether SAR Explorations: Reduction of the Aromatic Content and Additional Conformational Constraints through Methyl Substitution.
19.3. Structural studies
19.4. Conclusions
19.5. References
20. Solithromycin: Fourth Generation Macrolide Antibiotic
David Pereira, Sara Wu, Shingai Majuru, Stephen E. Schneider and Lovy Pradeep
20.1. Introduction
20.2. Structure-activity relationship of ketolides and selection of solithromycin
20.2.1. MIC testing of triazole analogs
20.2.2. Importance of 2-fluorine for activity
20.2.3. In vitro genotoxicity studies on solithromycin
20.2.4. Mouse PK and protection studies
20.3. Mechanism of action
20.3.1. Ribosome binding of antibiotics
20.3.2. Ribosome binding of solithromycin
20.3.3. Solithromycin protein inhibition
20.4. Overcoming the Ketek effect
20.5. Manufacture of solithromycin
20.6. Polymorphism
20.7. Pharmaceutical development
20.7.1. Capsule development
20.7.1.1. Capsule formulation development
20.7.1.2. Capsule manufacturing process
20.7.1.3. Capsule dissolution
20.7.2. Powder for oral suspension
20.7.3. Solithromycin for injection
20.7.3.1. The challenge of solithromycin i.v. formulation development
20.8. Clinical data
20.8.1. Phase 1 PK and bioavailability
20.8.2. Phases 2 and 3 trials
20.9. Summary
Index
Mark L. Peterson, PhD, is Chief Operating Officer and Corporate Secretary at Cyclenium Pharma, of which he is a member of the founding management / scientific team. He has over 25 years of experience in the biotechnology and pharmaceutical industries.