Computational Lithography
Wiley Series in Pure and Applied Optics (Band Nr. 1)
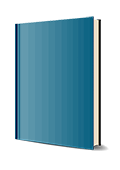
1. Auflage August 2010
256 Seiten, Hardcover
Wiley & Sons Ltd
Kurzbeschreibung
This is the first book to address the optimization of resolution enhancement techniques in optical lithography. It provides an in-depth discussion of RET tools that use model-based mathematical optimization approaches. The book starts with an introduction of optical lithography systems, electric magnetic field principles, and fundamentals of optimization; it goes on to describe algorithms for the development of optimal optical proximity correction, phaseshifting mask, offaxis illumination approaches, and their combinations. The accompanying mathematical derivations and MATLAB(r) software files make it easy for researchers, scientists, engineers, and graduate students and faculty to apply any of the optimization algorithms.
This is the first book to address the optimization of resolution enhancement techniques in optical lithography. It provides an in-depth discussion of RET tools that use model-based mathematical optimization approaches. The book starts with an introduction of optical lithography systems, electric magnetic field principles, and fundamentals of optimization; it goes on to describe algorithms for the development of optimal optical proximity correction, phaseshifting mask, offaxis illumination approaches, and their combinations. The accompanying mathematical derivations and MATLAB(r) software files make it easy for researchers, scientists, engineers, and graduate students and faculty to apply any of the optimization algorithms.
Acknowledgments.
Acronyms.
1 Introduction.
1.1 Optical Lithography.
1.1.1 Optical Lithography and Integrated Circuits.
1.1.2 Brief History of Optical Lithography Systems.
1.2 Rayleigh's Resolution.
1.3 Resist Processes and Characteristics.
1.4 Techniques in Computational Lithography.
1.4.1 Optical Proximity Correction.
1.4.2 Phase Shifting Masks.
1.4.3 Offaxis Illumination.
1.4.4 Second Generation RETs.
1.5 Outline.
2 Optical Lithography Systems.
2.1 Partially Coherent Imaging Systems.
2.1.1 Abbe's Model.
2.1.2 Hopkins Diffraction Model.
2.1.3 Coherent and Incoherent Imaging Systems.
2.2 Approximation Models.
2.2.1 Fourier Series Expansion Model.
2.2.2 Singular Value Decomposition Model.
2.2.3 Average Coherent Approximation Model.
2.2.4 Discussion and Comparison.
2.3 Summary.
3 Rule-based Resolution Enhancement Techniques.
3.1 RET Types.
3.1.1 Rule-based RETs.
3.1.2 Model-based RETs.
3.1.3 Hybrid RETs.
3.2 Rule-based OPC.
3.2.1 Catastrophic OPC.
3.2.2 One-dimensional OPC.
3.2.3 Line-shortening Reduction OPC.
3.2.4 Two-dimensional OPC.
3.3 Rule-based PSM.
3.3.1 Dark-field Application.
3.3.2 Light-field Application.
3.4 Rule-based OAI.
3.5 Summary.
4 Fundamentals of Optimization.
4.1 Definition and Classification.
4.1.1 Definitions in The Optimization Problem.
4.1.2 Classification of Optimization Problems.
4.2 Unconstrained Optimization.
4.2.1 Solution of Unconstrained Optimization Problem.
4.2.2 Unconstrained Optimization Algorithms.
4.3 Summary.
5 Computational Lithography with Coherent Illumination.
5.1 Problem Formulation.
5.2 OPC Optimization.
5.2.1 OPC Design Algorithm.
5.2.2 Simulations.
5.3 Two-phase PSM Optimization.
5.3.1 Two-phase PSM Design Algorithm.
5.3.2 Simulations.
5.4 Generalized PSM Optimization.
5.4.1 Generalized PSM Design Algorithm.
5.4.2 Simulations.
5.5 Resist Modeling Effects.
5.6 Summary.
6 Regularization Framework.
6.1 Discretization Penalty.
6.1.1 Discretization Penalty for OPC Optimization.
6.1.2 Discretization Penalty for Two-phase PSM Optimization.
6.1.3 Discretization Penalty for Generalized PSM Optimization.
6.2 Complexity Penalty.
6.2.1 Total Variation Penalty.
6.2.2 Global Wavelet Penalty.
6.2.3 Localized Wavelet Penalty.
6.3 Summary.
7 Computational Lithography with Partially Coherent Illumination.
7.1 OPC Optimization.
7.1.1 OPC Design Algorithm using the Fourier Series Expansion Model.
7.1.2 Simulations using the Fourier Series Expansion Model.
7.1.3 OPC Design Algorithm using the Average Coherent Approximation Model.
7.1.4 Simulations using the Average Coherent Approximation Model.
7.1.5 Discussion and Comparison.
7.2 PSM Optimization.
7.2.1 PSM Design Algorithm using the Singular Value Decomposition Model.
7.2.2 Discretization Regularization for PSM Design Algorithm.
7.2.3 Simulations.
7.3 Summary.
8 Other RET Optimization Techniques.
8.1 Double Patterning Method.
8.2 Post-Processing based on 2D DCT.
8.3 Photoresist Tone Reversing Method.
8.4 Summary.
9 Source and Mask Optimization.
9.1 Lithography Preliminaries.
9.2 Topological Constraint.
9.3 Source Mask Optimization Algorithm.
9.4 Simulations.
9.5 Summary.
10 Coherent Thickmask Optimization.
10.1 Kirchhoff Boundary Conditions.
10.2 Boundary Layer Model.
10.2.1 Boundary Layer Model in Coherent Imaging Systems.
10.2.2 Boundary Layer Model in Partially Coherent Imaging Systems.
10.3 Lithography Preliminaries.
10.4 OPC Optimization.
10.4.1 Topological Constraint.
10.4.2 OPC Optimization Algorithm based on BL Model under Coherent Illumination.
10.4.3 Simulations.
10.5 PSM Optimization.
10.5.1 Topological Constraint.
10.5.2 PSM Optimization Algorithm based on BL Model under Coherent Illumination.
10.5.3 Simulations.
10.6 Summary.
11 Conclusions and New Directions of Computational Lithography.
11.1 Conclusion.
11.2 New Directions of Computational Lithography.
Appendix A Formula derivation in Chapter 5.
Appendix B Manhattan geometry.
Appendix C Formula derivation in Chapter 6.
Appendix D Formula derivation in Chapter 7.
Appendix E Formula derivation in Chapter 8.
Appendix F Formula derivation in Chapter 9.
Appendix G Formula derivation in Chapter 10.
Appendix H Software Guide.
References.
Index.
Dr. Gonzalo R. Arce received a PhD degree in electrical engineering from Purdue University. He is the Charles Black Evans Distinguished Professor of Electrical and Computer Engineering at the University of Delaware and holds the Fulbright-Nokia Distinguished Chair in Information and Communications Technologies. Dr. Arce's fields of interest include nonlinear and statistical signal processing, digital printing, and computational imaging. He is a Fellow of the IEEE for his contributions to the theory and applications of nonlinear signal processing.