Photorefractive Materials for Dynamic Optical Recording
Fundamentals, Characterization, and Technology
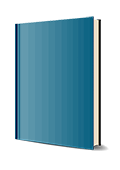
1. Auflage März 2020
352 Seiten, Hardcover
Wiley & Sons Ltd
A comprehensive and up-to-date reference on holographic recording
Photorefractive Materials for Dynamic Optical Recording offers a comprehensive overview of the physics, technology, and characterization of photorefractive materials that are used for optical recording. The author, a noted expert on the topic, offers an exploration of both transient and permanent holographic information storage methods. The text is written in clear terms with coherent explanations of the different methods that allows for easy access to the most appropriate method for a specific need.
The book provides an analysis of the fundamental properties of the materials and explores the dynamic recording of a spatial electric charge distribution and the associated spatial electric field distribution. The text also includes information on the characterization of photorefractive materials using holographic and nonholographic optical methods and electrical techniques, reporting a large number of actual experimental results on a variety of materials. This important resource:
* Offers an in-depth source of information on the physics and technology of all relevant holographic recording methods
* Contains text written by a pioneer in the field--Jaime Frejlich's research defined the field of dynamic holographic recording
* Presents a one-stop resource that covers all phenomena and methods
* Includes a review of the practical applications of the technology
Written for materials scientists, solid state physicists, optical physicists, physicists in industry, and engineering scientists, Photorefractive Materials for Dynamic Optical Recording offers a comprehensive resource on the topic from the groundbreaking expert in the field.
List of Tables xxxiii
Preface xxxv
Acknowledgments xxxvii
Part I Fundamentals 1
Introduction 3
1 Electro-Optic Effect 5
1.1 Light Propagation in Crystals 5
1.1.1 Wave Propagation in Anisotropic Media 5
1.1.2 General Wave Equation 6
1.1.3 Index Ellipsoid 6
1.2 Tensorial Analysis 8
1.3 Electro-Optic Effect 8
1.4 Perovskite Crystals 11
1.5 Sillenite Crystals 11
1.5.1 Index Ellipsoid 11
1.5.1.1 Index Ellipsoid with Applied Electric Field 13
1.5.2 Other Cubic Noncentrosymmetric Crystals 15
1.5.3 Lithium Niobate 15
1.5.4 KDP-(KH2PO4) 16
1.5.5 Bismuth Tellurium Oxide-Bi2TeO5 (BTeO) 17
1.6 Concluding Remarks 17
2 Photoactive Centers and Photoconductivity 19
2.1 Photoactive Centers: Deep and Shallow Traps 20
2.1.1 Cadmium Telluride 21
2.1.2 Sillenite-Type Crystals 22
2.1.2.1 Doped Sillenites 25
2.1.3 Lithium Niobate 28
2.1.4 Bismuth Telluride Oxide: Bi2TeO5 28
2.2 Luminescence 28
2.3 Photoconductivity 29
2.3.1 Localized States: Traps and Recombination Centers 29
2.3.2 Theoretical Models 32
2.3.2.1 One-Center Model 35
2.3.2.2 Two-Center/One-Charge Carrier Model 37
2.3.2.3 Dark Conductivity and Dopants 40
2.4 Photovoltaic Effect 40
2.4.1 Photovoltaic Crystals 41
2.4.1.1 Lithium Niobate and Other Ferroelectric Crystals 41
2.4.1.2 Some Photovoltaic Nonferroelectric Materials 41
2.4.2 Light Polarization-Dependent Photovoltaic Effect 43
2.5 Nonlinear Photovoltaic Effect 44
2.5.1 Light-Induced Absorption and Nonlinear Photovoltaic Effects 46
2.5.2 Deep and Shallow Centers 47
2.6 Light-Induced Absorption or Photochromic Effect 48
2.6.1 Transmittance with Light-Induced Absorption 51
2.7 Dember or Light-Induced Schottky Effect 51
2.7.1 Dember and Photovoltaic Effects 54
Part II Holographic Recording 55
Introduction 56
3 Recording a Space-Charge Electric Field 57
3.1 Index-of-Refraction Modulation 60
3.2 General Formulation 63
3.2.1 Rate Equations 64
3.2.2 Solution for Steady-State 64
3.3 First Spatial Harmonic Approximation 66
3.3.1 Steady-State Stationary Process 68
3.3.1.1 Diffraction Efficiency 69
3.3.1.2 Hologram Phase Shift 70
3.3.2 Time-Evolution Process: Constant Modulation 70
3.4 Steady-State Nonstationary Process: Running Holograms 72
3.4.1 Running Holograms with Hole-Electron Competition 76
3.4.1.1 Mathematical Model 78
3.5 Photovoltaic Materials 84
3.5.1 Uniform Illumination: N/ x = 0 84
3.5.2 Interference Pattern of Light 85
3.5.2.1 Influence of Donor Density 86
4 Volume Hologram with Wave Mixing 89
4.1 Coupled Wave Theory: Fixed Grating 89
4.1.1 Diffraction Efficiency 91
4.1.2 Out of Bragg Condition 91
4.2 Dynamic Coupled Wave Theory 92
4.2.1 Combined Phase-Amplitude Stationary Gratings 92
4.2.1.1 Fundamental Properties 94
4.2.1.2 Irradiance 95
4.2.2 Pure Phase Grating 96
4.2.2.1 Time Evolution 96
4.2.2.2 Stationary Hologram 100
4.2.2.3 Steady-State Nonstationary Hologram with Wave-Mixing and Bulk Absorption 106
4.2.2.4 Gain and Stability in Two-Wave Mixing 110
4.3 Phase Modulation 115
4.3.1 Phase Modulation in Dynamically Recorded Gratings 116
4.3.1.1 Phase Modulation in the Signal Beam 116
4.3.1.2 Output Phase Shift 118
4.4 Four-Wave Mixing 119
4.5 Conclusions 120
5 Anisotropic Diffraction 121
5.1 Coupled-Wave with Anisotropic Diffraction 121
5.2 Anisotropic Diffraction and Optical Activity 122
5.2.1 Diffraction Efficiency with Optical Activity, rho 123
5.2.2 Output Polarization Direction 123
6 Stabilized Holographic Recording 125
6.1 Introduction 125
6.2 Mathematical Formulation 127
6.2.1 Stabilized Stationary Recording 129
6.2.1.1 Stable Equilibrium Condition 130
6.2.2 Stabilized Recording of Running (Nonstationary) Holograms 130
6.2.2.1 Stable Equilibrium Condition 132
6.2.2.2 Speed of the Fringe-Locked Running Hologram 132
6.2.3 Self-Stabilized Recording with Arbitrarily Selected Phase Shift 133
6.3 Self-Stabilized Recording in Actual Materials 135
6.3.1 Self-Stabilized Recording in Sillenites 136
6.3.2 Self-Stabilized Recording in LiNbO3 136
6.3.2.1 Holographic Recording without Constraints 137
6.3.2.2 Self-Stabilized Recording 142
Part III Materials Characterization 151
Introduction 152
7 General Electrical and Optical Techniques 155
7.1 Electro-Optic Coefficient 155
7.2 Light-Induced Absorption 157
7.3 Dark Conductivity 161
7.4 Photoconductivity 162
7.4.1 Photoconductivity in Bulk Material 163
7.4.2 Alternating Current Technique 164
7.4.3 Wavelength-Resolved Photoconductivity 166
7.4.3.1 Transverse Configuration 166
7.4.3.2 Longitudinal Configuration 170
7.5 Photo-Electric Conversion 173
7.5.1 Wavelength-Resolved Photo-Electric Conversion (WRPC) 173
7.5.1.1 Undoped BTO 174
7.6 Modulated Photoconductivity 175
7.6.1 Quantum Efficiency and Mobility-Lifetime Product 176
7.7 Photo-Electromotive-Force Techniques (PEMF) 178
7.7.1 Speckle-Photo-Electromotive-Force (SPEMF) Techniques 178
7.7.1.1 Speckle Pattern onto a Photorefractive Material: Stationary State 179
8 Holographic Techniques 189
8.1 Holographic Recording and Erasing 189
8.2 Direct Holographic Techniques 189
8.2.1 Energy Coupling 190
8.2.2 Diffraction Efficiency 192
8.2.2.1 Debye Length Dependence on Light Intensity 193
8.2.3 Holographic Sensitivity 193
8.2.3.1 Computing S 195
8.3 Hologram Recording 195
8.4 Hologram Erasure 195
8.4.1 One Single Photoactive Center Involved 196
8.4.1.1 Bulk Absorption 196
8.4.2 Two (or More) Photoactive Centers (Localized States) Involved 197
8.4.2.1 Same Charge Carriers 197
8.4.2.2 Holes and Electrons on Different Photoactive Centers 197
8.5 Materials 197
8.5.1 Fe-doped LiNbO3: Hologram Erasure under White Light Illumination 197
8.5.2 Bi12TiO20 (BTO) 199
8.5.2.1 Undoped BTO under lambda = 780 nm Illumination 199
8.5.2.2 Bi12TiO20:Pb (BTO:Pb) 200
8.5.2.3 Bi12TiO20:V (BTO:V) 202
8.5.2.4 Holographic Relaxation in the Dark: Dark Conductivity 203
8.6 Phase Modulation Techniques 205
8.6.1 Holographic Sensitivity 205
8.6.2 Holographic Phase-Shift Measurement 206
8.6.2.1 Wave-Mixing Effects 207
8.6.3 Photorefractive Response Time 207
8.6.4 Selective Two-Wave Mixing 210
8.6.4.1 Amplitude and Phase Effects in GaAs 212
8.6.5 Running Holograms 214
8.7 Holographic Photo-Electromotive-Force (HPEMF) Techniques 218
9 Self-Stabilized Holographic Techniques 229
9.1 Holographic Phase Shift 229
9.2 Fringe-Locked Running Holograms 232
9.2.1 Absorbing Materials 232
9.2.1.1 Low Absorption Approximation 234
9.2.2 Characterization of Materials 234
9.2.2.1 Measurements 235
9.2.2.2 Theoretical Fitting 236
9.3 Characterization of LiNbO3:Fe 239
Part IV Applications 243
Introduction 244
10 Vibrations and Deformations 245
10.1 Measurement of Vibration and Deformation 245
10.2 Experimental Setup 246
10.2.1 Reading of Dynamic Holograms 247
10.2.2 Optimization of Illumination 247
10.2.2.1 Target Illumination 247
10.2.2.2 Distribution of Light among Reference and Object Beams 247
10.2.3 Self-Stabilization Feedback Loop 249
10.2.4 Vibrations 251
10.2.5 Deformation and Tilting 252
10.2.5.1 Applications of PEMF to Mechanical Vibration Measurements 256
11 Fixed Holograms 257
11.1 Introduction 257
11.2 Fixed Holograms in LiNbO3 257
11.2.1 Simultaneous Recording and Compensation 258
11.2.1.1 Theory 258
11.2.1.2 Experiment: Simultaneous Recording and Compensating 260
12 Photoelectric Conversion 263
12.1 Photoelectric Conversion Efficiency: Dember and Photovoltaic Effects 263
Part V Appendix 265
Introduction 266
Appendix A Reversible Real-Time Holograms 267
A.1 Naked-Eye Detection 267
A.1.1 Diffraction 267
A.1.2 Interference 268
A.2 Instrumental Detection 268
Appendix B Diffraction Efficiency Measurement 271
B.1 Angular Bragg Selectivity 271
B.1.1 In-Bragg Recording Beams 272
B.1.2 Probe Beam 272
B.2 Reversible Holograms 274
B.3 High Index-of-Refraction Material 275
Appendix C Effectively Applied Electric Field 279
Appendix D Physical Meaning of Some Parameters 281
D.1 Temperature 281
D.1.1 Debye Screening Length 282
D.1.1.1 Debye Length in Photorefractives 283
D.2 Diffusion and Mobility 284
Appendix E Photodiodes 287
E.1 Photovoltaic Regime 288
E.2 Photoconductive Regime 289
E.3 Operational Amplifier 290
Bibliography 291
Index 305