Aerodynamics of Wind Turbines
A Physical Basis for Analysis and Design
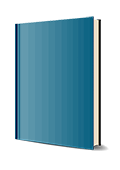
1. Edition August 2019
312 Pages, Softcover
Textbook
A review of the aerodynamics, design and analysis, and optimization of wind turbines, combined with the author's unique software
Aerodynamics of Wind Turbines is a comprehensive introduction to the aerodynamics, scaled design and analysis, and optimization of horizontal-axis wind turbines. The author -a noted expert on the topic - reviews the fundamentals and basic physics of wind turbines operating in the atmospheric boundary layer. He then explores more complex models that help in the aerodynamic analysis and design of turbine models. The text contains unique chapters on blade element momentum theory, airfoil aerodynamics, rotational augmentation, vortex-wake methods, actuator-line modeling, and designing aerodynamically scaled turbines for model-scale experiments. The author clearly demonstrates how effective analysis and design principles can be used in a wide variety of applications and operating conditions.
The book integrates the easy-to-use, hands-on XTurb design and analysis software that is available on a companion website for facilitating individual analyses and future studies. This component enhances the learning experience and helps with a deeper and more complete understanding of the subject matter. This important book:
* Covers aerodynamics, design and analysis and optimization of wind turbines
* Offers the author's XTurb design and analysis software that is available on a companion website for individual analyses and future studies
* Includes unique chapters on blade element momentum theory, airfoil aerodynamics, rotational augmentation, vortex-wake methods, actuator-line modeling, and designing aerodynamically scaled turbines for model-scale experiments
* Demonstrates how design principles can be applied to a variety of applications and operating conditions
Written for senior undergraduate and graduate students in wind energy as well as practicing engineers and scientists, Aerodynamics of Wind Turbines is an authoritative text that offers a guide to the fundamental principles, design and analysis of wind turbines.
Preface xv
Acknowledgments xvii
Abbreviations xix
List of Symbols xxi
About the Companion Website xxix
1 Introduction: Wind Turbines and the Wind Resource 1
1.1 A Brief History of Wind Turbine Development 1
1.1.1 Why "Wind Energy"? 1
1.1.2 Wind Turbines Then and Now 2
1.1.2.1 The Windmill - Hero of Alexandria (First Century CE) 2
1.1.2.2 1200s-1300s - Post Mills and Tower Mills 3
1.1.2.3 1700s - John Smeaton 3
1.1.2.4 1800s -Windmills in the American West 5
1.1.2.5 Late 1800s -Wind in Transition (Mechanical - Electricity, Drag - Aerodynamic Principles) 5
1.1.2.6 1900s-1950s -Wind Turbines across Scales (kW- MW) 6
1.1.2.7 1970s-2000s - Modern Utility-Scale Wind Turbines (>1MW) 7
1.1.3 Influence of Aerodynamics on Wind Turbine Development 8
1.1.4 Design Evolution of Modern Horizontal-Axis Wind Turbines 10
1.2 Wind Resource Characterization 11
1.2.1 Wind Resource - Available Power in the Wind 13
1.2.2 Basic Characteristics of the Atmospheric Boundary Layer 16
1.2.2.1 Steady Wind Speed Variation with Height 17
1.2.2.2 Turbulence and Stability State 19
1.2.2.3 Atmospheric Properties (Troposphere) 23
1.2.3 Statistical Description of Wind Data 24
1.2.3.1 Rayleigh Distribution 25
1.2.3.2 Weibull Distribution 26
1.2.4 Wind Energy Production Estimates 27
References 28
Further Reading 29
2 Momentum Theory 31
2.1 Actuator Disk Model 31
2.1.1 Basic Streamtube Analysis 31
2.1.2 Axial Induction Factor, a 34
2.1.3 Rotor Thrust and Power 35
2.1.4 Optimum Rotor Performance - The Betz Limit 35
2.1.5 Wake Expansion and Wake Shear 37
2.1.6 Validity of the Actuator Disk Model 38
2.1.7 Summary - Actuator Disk Model 39
2.2 Rotor Disk Model 40
2.2.1 Extended Streamtube Analysis 40
2.2.2 Angular Induction Factor, a' 42
2.2.3 Rotor Torque and Power 43
2.2.4 Optimum Rotor Performance Including Wake Rotation 44
2.2.5 Validity of the Rotor Disk Model 48
2.2.6 Summary - Rotor Disk Model 49
References 49
Further Reading 50
3 Blade Element Momentum Theory (BEMT) 51
3.1 The Blade Element - Incremental Torque and Thrust 51
3.1.1 Airfoil Nomenclature 52
3.1.2 Blade Element Velocity and Force/Torque Triangles 53
3.2 Combining Momentum Theory and Blade Element Theory through a, a', and Phi 55
3.2.1 Sectional Thrust and Torque in Momentum and Blade Element Theory 56
3.2.2 Rotor Thrust and Power in Blade Element Theory 56
3.3 Aerodynamic Design and Performance of an Ideal Rotor 57
3.3.1 The Ideal Rotor Without Wake Rotation 58
3.3.2 The Ideal Rotor with Wake Rotation 59
3.4 Tip and Root Loss Factors 62
3.4.1 Prandtl Blade Number Correction versus Glauert Tip Correction - Historical Perspective 62
3.4.2 A Total Tip-/Root Loss Correction 64
3.4.3 Limitations of Classical Tip-/Root Corrections 66
3.4.4 Modern Approaches to Tip Modeling 66
3.4.4.1 Correction of Normal-/Tangential Force Coefficients (Shen et al.) 67
3.4.4.2 Helical Model for Tip Loss (Branlard et al.) 67
3.4.4.3 Decambering Effect at Blade Tip (Sørensen et al.) 68
3.4.4.4 Extended Glauert Tip Correction Using a g Function (Schmitz and Maniaci 2016) 69
3.5 BEM Solution Method 71
3.5.1 A System of Two Equations for Two Unknowns, a and a' 71
3.5.2 Iterative BEM Solution Methodologies - Analyzing a Given Blade Design 72
3.5.2.1 Simultaneous Solution of a and a' 73
3.5.2.2 Root-Finding Method of Single Equation for Phi 74
3.5.3 Thrust Coefficient in the Turbulent Wake State, a > 0.4 75
3.5.3.1 Glauert Empirical Relation 76
3.5.3.2 1st-Order Approximation (Wilson, Burton) 77
3.5.3.3 2nd-Order Approximation (Buhl) 77
3.6 Simplified BEMT (Wilson and Lissaman 1974) 78
3.7 Effect of Design Parameters on Power Coefficient 80
3.7.1 Effect of Blade Number and Solidity 81
3.7.2 Effect of Profile Drag 82
3.7.3 Combined Effects of Blade Number, Solidity, and Profile Drag 82
3.7.4 Effects of Rotor Speed and Blade Pitch 84
3.7.5 Aerodynamic Considerations - Two Blades versus Three Blades 87
3.7.6 Analysis of a MW-Scale Pitch-/Speed-Controlled Wind Turbine 89
3.8 Validity of BEMT 97
3.8.1 Summary - BEMT 98
References 99
Further Reading 101
4 Wind Turbine Airfoils 103
4.1 Fundamentals of Airfoil Theory 103
4.1.1 Inviscid Flow: Thin-Airfoil Theory 105
4.1.1.1 Kutta-Joukowski Lift Theorem 106
4.1.1.2 Symmetric-/Cambered Thin Airfoil 106
4.1.1.3 Effect of Airfoil Thickness on Lift 110
4.1.1.4 d'Alembert's Paradox 111
4.1.2 Viscous Flow: Boundary-Layer Theory 111
4.1.2.1 Boundary-Layer Displacement Effect 113
4.1.2.2 Viscous Lift Theorem 115
4.1.2.3 Viscous Decambering Effect 117
4.1.2.4 Flow Separation and Stall 117
4.1.2.5 Understanding Profile Drag: Pressure and Skin Friction 119
4.1.2.6 Laminar-Turbulent Transition 120
4.2 Design Characteristics of Wind Turbine Airfoils 122
4.2.1 Radial Variation of the Reynolds Number 122
4.2.2 Force/Torque and Velocity Triangle Along the Blade Radius 123
4.2.3 Airfoil Design Criteria for Wind Turbine Blades 124
4.3 Development of Wind Turbine Airfoils 126
4.3.1 A Brief Historical Review of Wind Turbine Airfoils 126
4.3.2 Catalog of Wind Turbine Airfoils 129
References 133
Further Reading 136
5 Unsteady Aerodynamics and 3-D Correction Models for Airfoil Characteristics 137
5.1 Unsteady Aerodynamics on Wind Turbine Blades 137
5.1.1 Fundamentals of Unsteady Aerodynamics - Theodorsen's Theory 138
5.1.1.1 Flow Model - Unsteady Thin-Airfoil Theory 139
5.1.1.2 Special Case: Freestream Angle-of-Attack Oscillation 140
5.1.2 Dynamic Stall Models 141
5.1.3 Relevance of Atmospheric Boundary Layer on Unsteady Aerodynamics 143
5.1.3.1 Effect of Yawed Inflow, Mean Shear, and Tower Interaction 144
5.1.3.2 Effect of Atmospheric Turbulence 146
5.2 Rotational Augmentation and Stall Delay 148
5.2.1 Himmelskamp Effect 148
5.2.2 Coriolis Effect and Centrifugal Pumping 149
5.2.2.1 Coriolis Effect 149
5.2.2.2 Centrifugal Pumping 151
5.2.3 Stall Delay Models 152
5.2.3.1 Snel et al. 153
5.2.3.2 Corrigan and Schillings 153
5.2.3.3 Du and Selig 153
5.2.3.4 Chaviaropoulos and Hansen 154
5.2.3.5 Dumitrescu et al. 154
5.2.3.6 Eggers et al. 155
5.2.3.7 Lindenburg 155
5.2.3.8 Dowler and Schmitz 155
5.2.4 Scaling Rotational Augmentation from Small-Scale to Utility-Scale Turbines 158
5.2.5 Extraction of Rotational Augmentation Data from Computed Flow Fields 161
5.3 Airfoil Characteristics at High Angles of Attack 162
5.3.1 Flat-Plate Correction 163
5.3.2 Viterna-Corrigan Correction 163
5.3.3 Comments on High Angle-of-Attack Corrections 164
References 164
Further Reading 169
6 Vortex Wake Methods 171
6.1 Fundamentals of Prandtl Lifting-Line Theory 171
6.1.1 Vortex Sheet and Horseshoe Vortices 171
6.1.2 Inviscid Flow: Lifting-Line Theory 174
6.1.2.1 Elliptic Loading (Inviscid Airfoil Polar) 176
6.1.2.2 Parked NREL Phase VI Rotor (Viscous Airfoil Polar) 178
6.1.2.3 Parked NREL 5-MW Turbine - Optimum Blade Pitch in Low-/High Winds 182
6.2 Prescribed-Wake Methods 182
6.2.1 Helicoidal Vortex Filaments 183
6.2.2 Vortex-Sheet Geometry 184
6.2.3 Biot-Savart Law 186
6.2.4 Induced Velocities and Influence Coefficients 187
6.2.5 Relationship Between Vortex Theory and Blade-Element Theory 188
6.2.5.1 Sectional Thrust and Torque in Vortex Theory 189
6.2.5.2 Rotor Thrust and Power in Vortex Theory 190
6.2.6 Iterative Prescribed-Wake Solution Methodology 190
6.2.6.1 Krogstad Turbine - Prescribed-Wake versus BEM Solution Method 193
6.2.7 Limitations of Prescribed-Wake Methods 194
6.3 Free-Wake Methods 195
6.3.1 Trailing Vortices versus Shed Vortices 196
6.3.2 Lagrangian Markers and Blade Model 196
6.3.3 Iterative Free-Wake Solution Methodology 199
6.3.4 Handling Singularities - Viscous Core Models 200
6.3.4.1 Vortex Stretching 200
6.3.4.2 Rankine Vortex 201
6.3.4.3 Lamb-Oseen Vortex 201
6.3.4.4 Difficulties of Viscous Core Models 202
6.3.5 Singularity-Free-Wake - Distributed Vorticity Elements (DVEs) 202
6.3.5.1 The Multi-Lifting-Line Method of Horstmann 203
6.3.5.2 The Singularity-Free-Wake Method of Bramesfeld and Maughmer 203
6.3.6 Prediction of Blade Tip Loads - Free-Wake versus Prescribed-Wake/BEM Methods 204
6.3.7 Limitations of Free-Wake Methods 205
References 205
Further Reading 208
7 Advanced Computational Methods 209
7.1 High-Fidelity Blade-Resolved CFD Solutions 209
7.1.1 Unsteady Reynolds-Averaged Navier-Stokes Equations 210
7.1.2 Turbulence Modeling 211
7.1.2.1 k-epsilon Turbulence Model 211
7.1.2.2 k-omega Turbulence Model 212
7.1.2.3 Shear-Stress Transport (SST) k-omega-Based Turbulence Model 212
7.1.3 Effect of Laminar-/Turbulent Transition on CFD Predictions 213
7.1.4 Coupling of Navier-Stokes Solver with Helicoidal Vortex Model 214
7.2 Numerical Modeling of Wind Turbine Wakes 217
7.2.1 Engineering-Type Wake Models 217
7.2.2 Actuator Wake Models 218
7.2.2.1 ALM - Actuator-Line Model (Sørensen and Shen) 220
7.2.2.2 ALM* - Variable-epsilon Actuator-Line Model 220
7.2.2.3 ACE - Actuator Curve Embedding (Jha and Schmitz) 222
7.2.3 Limitations of Actuator Methods 225
7.3 Wake Modeling - Effect of Atmospheric Stability State 226
7.3.1 Atmospheric Boundary Layer LES Solver in OpenFOAM 227
7.3.2 Example of Turbine-Turbine Interaction for Neutral/Unstable Stability 229
7.3.3 Effect of ALM Approach on Wind Turbine Array Performance Prediction 230
7.3.4 Bridging the Gap - Meso-Microscale Coupling 231
References 233
Further Reading 239
8 Design Principles, Scaled Design, and Optimization 241
8.1 Design Principles for Horizontal-Axis Wind Turbines 241
8.1.1 Wind Turbine Design Standards 242
8.1.1.1 IEC Standards for Wind Turbines 243
8.1.1.2 Wind Turbine Design Loads 243
8.1.2 Rotor Design Procedure 245
8.1.2.1 General Rotor Design Process 245
8.1.2.2 COE versus Levelized Cost of Energy (LCOE) 248
8.1.2.3 Computational Tools for Rotor Analysis and Design 249
8.2 Scaled Design of Wind Turbine Blades 250
8.2.1 Limitations of Scaled Blade Aerodynamics and Dynamics 251
8.2.2 Example of Scaled Aerodynamics from Utility-Scale to MS Turbine 252
8.2.2.1 Scaled Design with Given cl (Lift Coefficient) Distribution (Scaled NREL 5-MW) 256
8.2.2.2 Scaled Design with Given c (Chord) Distribution (PScaled NREL 5-MW) 257
8.2.2.3 Scaled Design with Given beta (Pitch/Twist) Distribution (TScaled NREL 5-MW) 258
8.2.2.4 Differences in Scaled Designs w.r.t. Airfoil Aerodynamics and Blade Loads 259
8.2.3 Model-Scale Wind Turbine Aerodynamics Experiments 261
8.2.3.1 NREL Phase VI Rotor 262
8.2.3.2 MEXICO Rotor 264
8.2.3.3 Krogstad Turbine 265
8.3 Aerodynamic Optimization of Wind Turbine Blades 267
8.3.1 Principles of Blade Element Momentum (BEM) Aerodynamic Design 268
8.3.1.1 Betz Optimum Rotor (Ideal Rotor Without Wake Rotation) 268
8.3.1.2 Effect of Rotation on BEM Optimum Blade Design 269
8.3.1.3 Effect of Profile Drag on BEM Optimum Blade Design 270
8.3.1.4 Effect of Root-/Tip Loss on BEM Optimum Blade Design 271
8.3.1.5 Limitations of BEM Aerodynamic Optimization 272
8.3.2 Principles of VWM Aerodynamic Design 273
8.3.2.1 Optimum Circulation Distribution Under Thrust Constraint 274
8.3.2.2 Betz Minimum Energy Condition 276
8.3.2.3 Effect of Profile Drag on VWM Optimum Blade Design (DTU 10-MW RWT) 279
8.3.2.4 Design of Large-Scale Offshore "Low Induction Rotor" (LIR) 284
8.3.2.5 Limitations of VWM Aerodynamic Optimization 289
8.4 Summary - Scaled Design and Optimization 290
References 291
Further Reading 294
Index 295